There are very valid reasons to focus on the potential of these "urban mines", also known as secondary mines, to distinguish them from "primary mines" where resources in the ground are exploited directly.
These alternative resources would not only address a shortage of mining infrastructure, but could also help to drastically reduce electronic waste, known as "e-waste".
The world's fastest growing waste stream, e-waste wreaks havoc on global ecosystems and poses a major health threat by releasing toxic substances into soil and water, especially in Asia.
Improving the recycling of electronic products could also reduce the high environmental impact of mining. In fact, for some metals, recycling is more energy-efficient than mining. For example, extracting aluminum through recycling requires 10 to 15 times less energy than primary production.
The issue is especially important as several of the recyclable metals are critical resources for the European Union's dual transitions to a digital and carbon-neutral economy.
Deposits such as lithium, cobalt, nickel and rare earths are essential for the production of electronic products, electric vehicles and renewable energy components such as solar panels.
However, they are little explored and exposed to a high risk of supply tensions. In this regard, every three years since 2011, the European Union has evaluated and published a list of critical raw minerals that should be a priority for urban mining.
The fifth list, published in 2023, identified 34 critical metals, including rare earth elements, lithium, copper and nickel. Unfortunately, the gap between the European Union's recommendations and urban mining practices is glaringly obvious.
A life cycle full of obstacles to recycling
The recovery potential of an object is limited at every stage of its life cycle by technical, organizational, regulatory and economic obstacles.
From their very design, certain practices limit the recyclability of their metals, such as the use of metals in specific alloys, since not all alloys can be recycled, or hybridization, since composite materials are more difficult - not impossible - to recycle.
For example, for liquid food packaging, most boxes are made of cardboard and PolyAl, a mixture of aluminum and polyethylene (a type of plastic).
For many years, cardboard from food packaging was recovered and recycled, but not PolyAl, leading to incomplete recycling.
In this specific case, companies such as Tetra Pak and Recon Polymers eventually developed a separation process, opening a recycling plant specifically for PolyAl in 2021.
But many other products remain difficult to recycle, precisely because this aspect was not considered at the design stage.
Dispersive uses, which involve using small amounts of metals in products to modify their properties, are another practice that escapes recycling.
In the case of silver nanoparticles, their industrial application ranges from disinfecting medical equipment and water treatment to preventing odors in textiles.
Similarly, a few grams of dysprosium, a rare earth metal, can also be used to increase the strength of magnets. In short, some metals have so many applications that it is impossible to guarantee their roundness.
Electronic hibernation - leaving our devices in the attic
Once the objects have been designed and used, there is a second obstacle, which comes from consumers, who tend to keep their electronic objects, whether they work or not, rather than handing them over to a specific recycling facility.
This phenomenon is known as electronic hibernation.
Back in 2009, a pioneering study estimated that American homes stored an average of 6.5 electronic products hibernating in their attics and basements. This number has increased exponentially over the years.
In 2021, a study conducted by Google identified seven main barriers that prevent consumers from recycling their electronic devices:
Low awareness of existing delivery options (recycling)
- Low awareness of existing delivery options (recycling)
- Expectations of financial or social compensation
- Device nostalgia
- Desire to keep spare products
- Data recovery factors
- Desire to guarantee data removal
- Inconvenience of delivery options.
A more recent study conducted in Switzerland slightly mitigates these results: 40% of respondents said they would be willing to part with their old phone for less than five dollars. However, it would be interesting to conduct the same survey in countries less wealthy than Switzerland.
Finally, the third obstacle concerns collection systems and recycling infrastructures. In France, the majority of targeted waste streams (e-waste, packaging, tires, etc.) are managed by eco-organizations, private bodies that have organizational or financial responsibility.
These are regularly embroiled in controversy: analyses indicate that the material recovery of waste streams managed by eco-organizations is often sub-optimal, in particular due to their profitability objectives.
Involving engineers, designers, politicians and consumers
Despite these obstacles, several initiatives aim to support companies in their eco-design efforts, including the cradle-to-cradle concept, which encourages companies to maintain "the quality of raw materials throughout the multiple life cycles of the product and its components".
Beyond these schemes, however, each participant in the value chain needs to examine their responsibility for waste:
For engineers and product designers, this means adopting a more sustainable approach to design, taking into account the entire life cycle of the product from the very beginning of the design phase: this is the purpose of eco-design and eco-conception.
Companies, for their part, need to adopt a long-term approach rather than focusing exclusively on short-term profitability, particularly in a context of volatile metal prices.
For consumers, this means a greater awareness of the need to separate waste for disposal in specific channels, particularly e-waste.
And finally, governments and local authorities would do well to implement regulations adapted to the complexity of the sector, potentially including ambitious targets for specific recycling rates per type of metal, as well as some kind of territorial planning to better coordinate flows.
Ensuring that recycling facilities are more accessible is also a key factor in promoting good recycling behavior.
The difficulty of moving towards a circular economy
We haven't yet ventured to report on metal recycling rates. One of them, the end-of-life recycling rate (EOL-RR), refers to the percentage of discarded metal that is recycled. Another indicator, recycled content (RC), considers the proportion of recycled metal in total metal production.
Unsurprisingly, these two indicators give very different recycling rates. For example, chromium (Cr), copper (Cu) and zinc (Zn) have a lifetime recycling rate of over 50%, which means that more than half of the quantities put into circulation are recycled.
However, their recycled content is between 10% and 25%, since the primary extraction of these metals is constantly increasing: the share of recycled metal in total flows therefore remains low.
Consequently, even if we managed to achieve optimal exploitation of urban mine deposits and high recycling rates for all metals (measured in EOL-RR), we would still be far from a circular economy, as demand for metals continues to increase exponentially.
For example, global copper (Cu) production has almost doubled since 2000, from 14 to 25 million metric tons/year.
Therefore, the effective recycling of metals contained in urban mines is a necessary but not sufficient condition for a true circular economy.
It will be necessary to see a significant decrease in the volume of mineral resources used in industry before urban mining can partially replace, rather than add to, the exploitation of primary deposits.
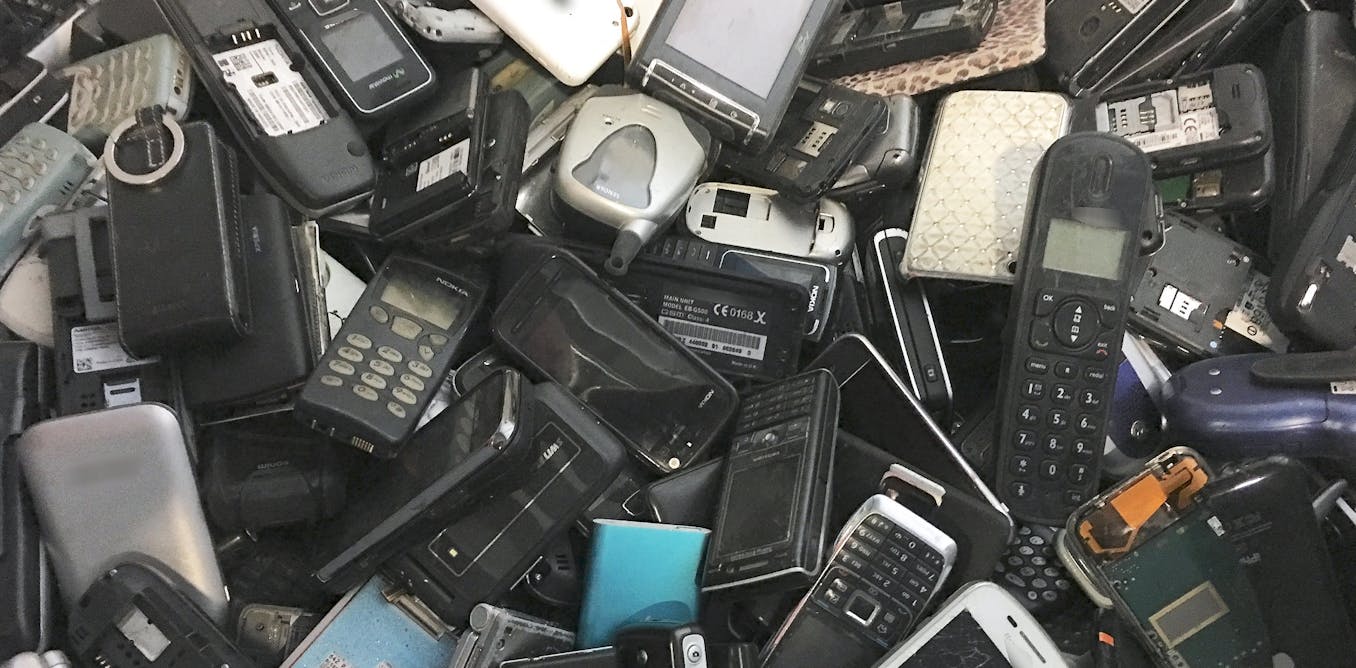